Article
Could SAI Delay the Recovery of the Ozone Layer?
The ozone layer that protects the Earth from harmful ultraviolet rays is slowly recovering from the damage done to it by human activity in the late 20th century. Stratospheric aerosol injection (SAI), an idea to cool the earth by adding tiny, reflective particles to the stratosphere which hosts the ozone layer, may slow this recovery even further.
Key takeaways
- Damage to the stratospheric ozone layer, which was caused by chlorofluorocarbon (CFC) pollutants, was first detected in the 1970s.
- International efforts to reduce emissions of CFCs have been successful, but due to their long lifetime in the atmosphere, the “hole” in the ozone layer is only slowly recovering.
- SAI would affect the chemistry of the stratosphere, with impacts on the ozone layer that could delay the recovery of the ozone hole by decades.
The Ozone layer
Ozone is present throughout the atmosphere, but the highest concentrations are in the stratosphere. This ozone layer filters out most of the harmful UV-B and UV-C radiation from the sun.
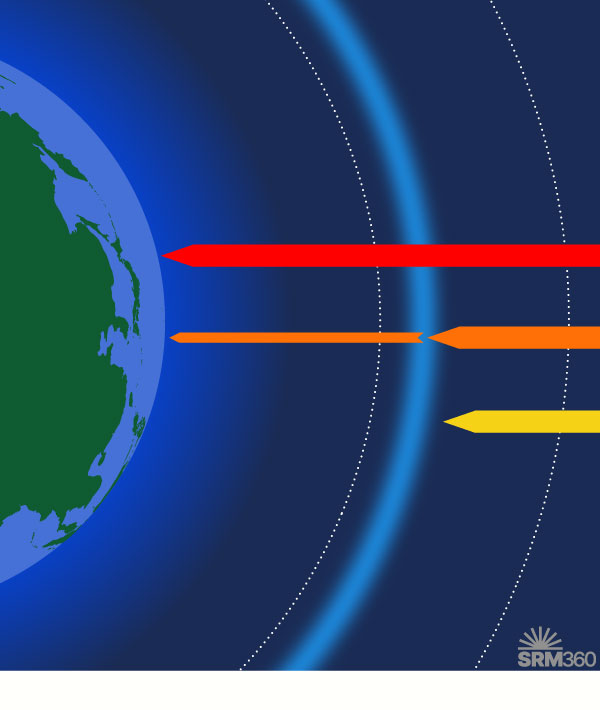
The ozone layer
UV-A
UV-B
Earth
UV-C
Troposphere
Stratosphere
Source: NASA
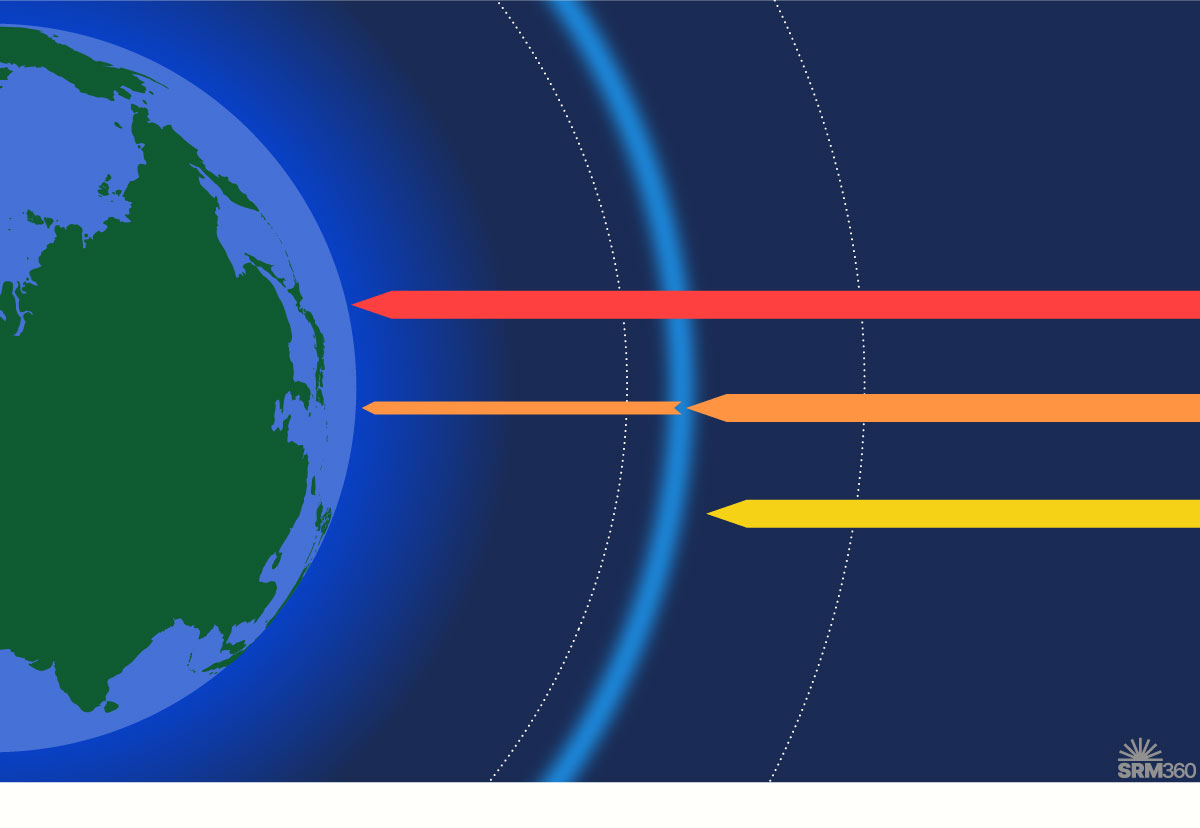
The ozone layer
UV-A
UV-B
Earth
UV-C
Troposphere
Stratosphere
Source: NASA
Ozone is a molecule that occurs naturally in the stratosphere – the layer of the atmosphere 10–50 km above the Earth’s surface. The ozone layer blocks some of the sun’s most harmful ultraviolet radiation (UV-B and UV-C) from reaching the Earth’s surface. Damage to the ozone layer has impacts on human and ecosystem health, including increased rates of cataracts (clouding of the eye’s lens) and skin cancer, and disruption of the marine food chain.
Stratospheric ozone depletion – or the “ozone hole” – is the result of human activity, but international cooperation has led to changes that have reduced the damage.
SAI is the most commonly studied sunlight reflection method – a set of hypothetical technologies that could counteract rising temperatures by reflecting more sunlight away from the Earth’s surface.
Models suggest that SAI could cool the planet in a similar way to large volcanic eruptions that release sulphur dioxide (SO2) into the atmosphere. The SO2 reacts with other molecules in the atmosphere to form tiny sulphate particles that reflect incoming sunlight and persist in the stratosphere for one to two years.
However, implementing SAI would also affect stratospheric chemistry, with potential impacts on the recovery of the ozone layer.1 The extent of these impacts is uncertain. Studies suggest the impacts would depend on factors such as the chemical composition of deployed aerosols and the geographic location of deployment.
How humans have damaged the ozone layer
Chlorofluorocarbons (CFCs) – a group of chemicals once commonly used in packaging, solvent cans, and refrigeration – have damaged the ozone layer. Though these chemicals are not toxic and persist for a long time in the lower atmosphere, they undergo rapid transformation once they enter the stratosphere and destroy ozone.2
Ozone destruction primarily occurs on the surfaces of polar stratospheric clouds, which form at extremely cold temperatures (<−78°C), mostly over Antarctica during winter in the Southern Hemisphere. CFCs become concentrated on the surfaces of these clouds, where they form reactive compounds that break down ozone when sunlight reappears in polar spring.3
Strong winds circle around Antarctica in the Southern Hemisphere winter, trapping the atmosphere in a polar vortex – a large region of rotating air. These strong winds persist into spring, enhancing polar stratospheric cloud formation, and increasing ozone depletion. This ozone-depleted part of the stratosphere is called the “ozone hole”.
Once the winds weaken at the end of spring, air masses with depleted ozone concentrations mix into the rest of the stratosphere. This leads to ozone thinning and increased UV levels across the entire world, but particularly in Southern Hemisphere countries such as Australia and New Zealand.
International action averted environmental catastrophe
Scientists began monitoring stratospheric ozone over Antarctica in 1956. By the 1980s, it became clear that ozone concentrations were decreasing, and CFCs were responsible.3
International response to ozone depletion began in the 1970s with the United Nations Environment Programme (UNEP) calling for intensive research and monitoring. The Montreal Protocol, which called for phasing out ozone-depleting chemicals, was adopted in September 1987.
The Montreal Protocol brought together representatives from industry, environmental groups, and governments. It was described by former UN Secretary-General Kofi Annan as “perhaps the single most successful international agreement to date” in 2005.
Since 1987, ozone-depleting chemicals have been phased out of production globally. However, CFCs have a very long lifetime in the atmosphere and small amounts of CFCs continue to be emitted – mostly from old equipment.4 The hole in the ozone layer reappears each polar spring, but it continues to shrink as CFCs become less abundant in the atmosphere.

Maldives representative signing Montreal Protocol.
(Photo: United Nations)How the ozone hole interacts with climate change
Damage to the ozone layer does not cause climate change. However, climate change can exacerbate ozone depletion.5
Warming in the lower atmosphere is linked with cooling in the stratosphere.6 This enhances polar stratospheric cloud formation and the strength of the polar vortex, which can increase ozone depletion.7
Although climate change can exacerbate ozone depletion, the Montreal Protocol has had its own unintended impact on climate change. Hydrofluorocarbons, adopted to replace CFCs, are powerful greenhouse gases.8 In 2016, an amendment to the Montreal Protocol phased out their production, which has reduced their impact on rising temperatures.
Could SAI interfere with ozone recovery?
Studies suggest that SAI’s impacts on stratospheric chemistry could have impacts on ozone recovery. Some models have shown that ozone concentrations would increase if sulphates were to be injected at mid-latitudes in the Northern Hemisphere.9
In polar regions, however, increasing stratospheric aerosol concentrations also increases the surface area for ozone-depleting reactions. In this way, injected aerosols would behave like polar stratospheric clouds and become the sites of ozone depletion, leading to decreased ozone in spring over Antarctica.1
Recent research suggests that SAI using non-sulphate particles to reflect sunlight could have a different impact on stratospheric ozone.10 For example, alumina particles would decrease ozone in a similar way to sulphates,11 but calcite particles might avoid or limit this effect.10 However, research on non-sulphate particles for SAI is limited.
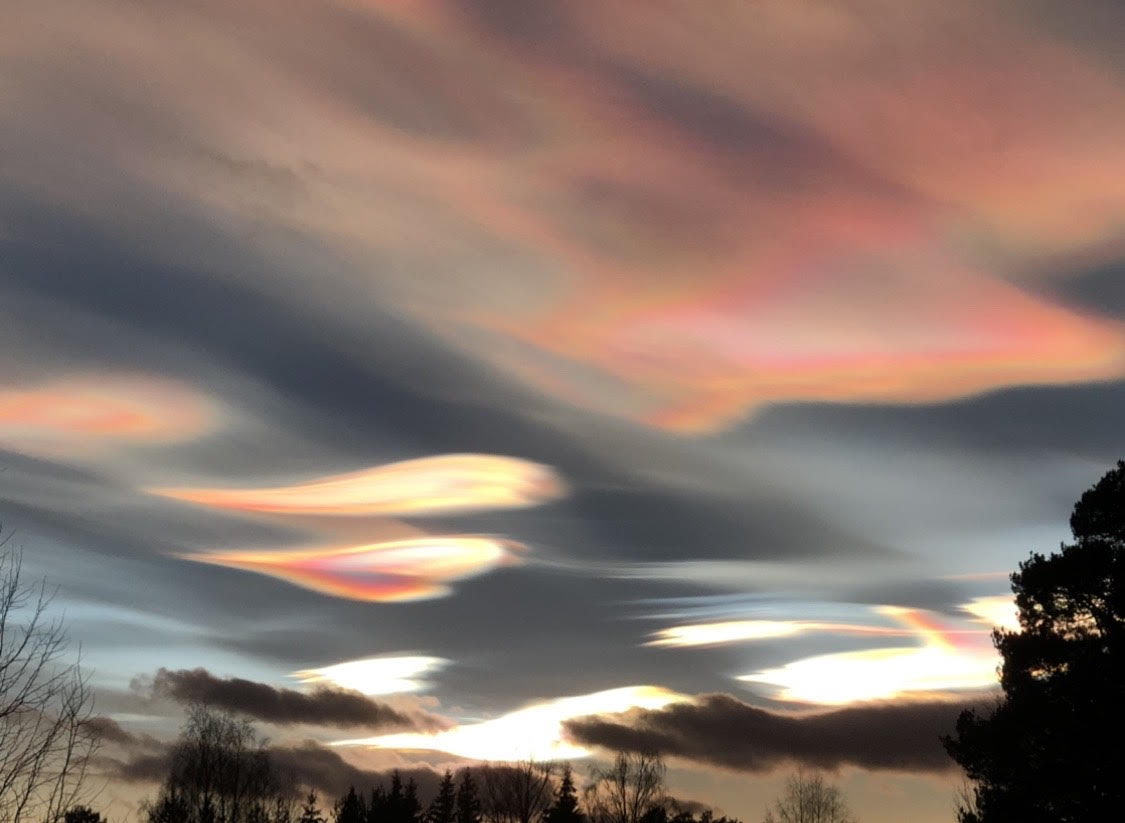
Polar stratospheric clouds that provide the crucial surface needed for ozone destroying chemical reactions to occur. The clouds pictured are in Norway, though they are rarer in the Arctic than in the much colder Antarctica, where they form every year.
A slow recovery of the ozone hole, made slower?
The international community has worked together for more than 50 years to address the issue of stratospheric ozone depletion. Efforts to eliminate ozone-depleting chemicals in the atmosphere have been largely successful and the ozone hole is slowly recovering.
Whether, and to what extent, SAI could undermine the recovery of the ozone layer is unclear, and the issue is being monitored by the Scientific Assessment Panel of the Montreal Protocol. International assessments suggest SAI using sulphates could delay ozone recovery by decades but would not lead to ozone depletion of the same magnitude as the late 20th century.1
Open questions
- How significant would the risks of ozone depletion from sulphate SAI be compared to the risks of climate change it could help reduce?
- Could SAI with sulphates be deployed strategically in a way that minimises its impact on ozone recovery?
- Could alternative aerosols for SAI cool the planet without undermining ozone recovery?
Endnotes
- Haywood J, Tilmes S, Keutsch F, et al. (2022) Chapter 6: Stratospheric Aerosol injection and its Potential Effect on the Stratospheric Ozone Layer. In: Scientific Assessment of Ozone Depletion 2022. pp. 325–375. https://csl.noaa.gov/assessments/ozone/2022/downloads/Chapter6_2022OzoneAssessment.pdf
- Molina MJ, Rowland FS (1974). Stratospheric sink for chlorofluoromethanes: Chlorine atomic-atalysed destruction of ozone. Nature; 249: 810–812. https://doi.org/10.1038/249810a0
- Toon OB, Turco RP (1991). Polar Stratospheric Clouds and Ozone Depletion. Sci Am; 264: 68–75. https://www.jstor.org/stable/24936942
- Lickley MJ, Daniel JS, Fleming EL, et al. (2022). Bayesian assessment of chlorofluorocarbon (CFC), hydrochlorofluorocarbon (HCFC) and halon banks suggest large reservoirs still present in old equipment. Atmos Chem Phys; 22: 11125–11136. https://doi.org/10.5194/acp-22-11125-2022
- Some models have predicted that cooler stratospheric temperatures would cause a “super recovery” of stratospheric ozone especially if greenhouse gas emissions are high.1 However, this modelled recovery is not uniform across the stratosphere and is complicated by other chemical reactions that could prevent “super recovery” of ozone in the upper stratosphere over Antarctica.12
- Hartmann DL, Wallace JM, Limpasuvan V, et al. (2000). Can ozone depletion and global warming interact to produce rapid climate change? PNAS; 97: 1412–1417. https://doi.org/10.1073/pnas.97.4.1412
- Tabazadeh A, Drdla K, Schoeberl MR, et al. (2002). Arctic “ozone hole” in a cold volcanic stratosphere. PNAS; 99: 2609–2612. https://doi.org/10.1073/pnas.052518199
- Molina M, Zaelke D, Sarma KM, et al. (2009). Reducing abrupt climate change risk using the Montreal Protocol and other regulatory actions to complement cuts in CO2 emissions. PNAS; 106: 20616–20621. https://doi.org/10.1073/pnas.0902568106
- Bednarz EM, Butler AH, Visioni D, et al. (2023) Injection strategy – a driver of atmospheric circulation and ozone response to stratospheric aerosol geoengineering. Atmos Chem Phys; 23: 13665–13684. https://doi.org/10.5194/acp-23-13665-2023
- Keith DW, Weisenstein DK, Dykema JA, et al. (2016). Stratospheric solar geoengineering without ozone loss. Proc Natl Acad Sci USA; 113: 14910–14914. https://doi.org/10.1073/pnas.1615572113
- Vattioni S, Luo B, Feinberg A, et al. (2023). Chemical Impact of Stratospheric Alumina Particle Injection for Solar Radiation Modification and Related Uncertainties. Geophys Res Lett; 50. DOI: 10.1029/2023GL105889. https://doi.org/10.1029/2023GL105889
- Maliniemi V, Nesse Tyssøy H, Smith-Johnsen C, et al. (2021). Effects of enhanced downwelling of NOx on Antarctic upper-stratospheric ozone in the 21st century. Atmos Chem Phys; 21: 11041–11052. https://doi.org/10.5194/acp-21-11041-2021